Darkness
How do you feel about the dark?
Do you perceive darkness to be a time of doom and gloom or a time to rest?
Some people associate darkness with evil, wickedness, corruption, sin, sinfulness, iniquity, immorality, devilry, etc. Others associate darkness to unhappiness or even secrecy. Or do you see it as a time of self-reflection and/or rest.
How you perceive darkness depends upon your religious beliefs, knowledge and life experiences. We all have dark periods in our life, but as they say: “Every dark cloud has a silver lining” and/or “See the light at the end of the tunnel”. Mankind as a rule seeks the light and enlightenment for it gives us hope and encouragement.
Through our periods of darkness we can learn from our mistakes, we can prepare ourselves to lead a better life and perhaps be a glowing example to others. But no one in their right mind would consider suffering an eon of darkness, however our universe did just that.
How The Dark Age Began
About 47,000 years after the Big Bang, [13.798953 Billion years ago (Bya)], the early universe started to decelerate at a faster rate. This brought on the beginning of the gravitational collapse of the early universe, about 70,000 years after the Big Bang, (13.79893 Bya). The early universe still consisted of 75% hydrogen and 25% helium and its distribution remained constant as the electron-baryon plasma continued to thin. The gravitational collapse was a very slow process.
It is thought that 377,000 years after the Big Bang, (13.798623 Bya), the temperature of the early universe fell to about 4,000 K (Kelvin). This brought on the era of Recombination that lasted for about 3 million years. About 380,000 years after the Big Bang, (13.79862 Bya), the Cosmic Microwave Background (CMB) created the Afterglow Light Pattern that is still detectable today.
About 400,000 years after the Big Bang, (13.7986 Bya), Density Waves began to imprint their characteristic polarization (wave) signals through out the early universe.
The decoupled photons of the Recombination era probably would have filled the universe with a brilliant pale orange glow at first and then gradually red shifted to non-visible wavelengths after about 3 million years leaving it without visible light. The end of the “Recombination” era brought on the Dark Age.
The Dark Age
Although it is thought that the Dark Age lasted up to about 1 billion years after the Big Bang, it began to change about 400 million years after the Big Bang. So! Somewhere between 3.377 million years after the Big Bang (13.795623 Bya) and 1 billion years after the Big Bang (12.799 Bya), the Dark Age took place.
However, the early universe was not meant to die, it struggled and kept changing.
About 10 million years after the Big Bang (13.789 Bya) traces of heavy elements began to develop. These are the heavy elements whose later chemical reactions would spark the beginning of life.
It is believed that between 10 to 17 million years after the Big Bang, (13.789 to 13.782 Bya), the temperature of cosmic background radiation cooled from some 4,000 K (Kelvin) down to about 60 K. The background temperature of the early universe was between 373 K and 273 K, this would have allowed the possibility of liquid water to form.
About 100 million years after the Big Bang (13.699 Bya) the Gravitational collapse of ordinary matter particles started to fall into structures created by dark matter. Quasars begin to take shape as the gaseous accretion forms disks around black holes. As the gas falls toward a black hole, energy is released in the form of electromagnetic radiation. Population III (Pop III) stars were starting to form. They were very hot and had a short lifetime. Their ultraviolet light started to ionize the remaining neutral hydrogen gas.
Population III Stars are a hypothetical population of extremely massive and hot stars with virtually no metals, except possibly for intermixing ejecta from other nearby Population III supernovas. It is thought that they were composed entirely of the primordial gas such as hydrogen, helium and very small amounts of lithium and beryllium. They have been observed in the quasar emission spectra and are also thought to be components of faint blue galaxies. But their existence is inferred from physical cosmology, they have not yet been observed directly.
The Reionization era began about 150 million years after the Big Bang (13.649 Bya). It is thought that the supernovae of the Population III, (Pop III), stars was the possible mechanism for the reionization. The end of the Reionization era was also the end of the Dark Ages. It ended about 1 billion years after the Big Bang.
Between 150 and 200 million years after the Big Bang (13.649 to 13.599 Bya) the Population II (Pop II) stars started to form.
Population II Stars were presumably created from interstellar gas clouds that emerged shortly after the big bang. They are relatively rich in hydrogen and helium but are poor in elements heavier than helium, containing 10 to 100 times less of these elements than Population I stars. Population II stars are mainly found in Globular clusters and the halo of both spiral and elliptical galaxies. Some are found in the bulge of galaxies. Those found in the galactic halo are older and thus more metal poor.
As the reionization intensifies, photons of light scatter off free protons and electrons, hence the early Universe becomes opaque again.
About 200 million years after the Big Bang (13.599 Bya) HD 140283, (the “Methuselah” Star), formed. Methuselah is a metal-poor sub-giant star about 200 light years away from the Earth in the constellation Libra, specifically towards the Ophiuchus constellation. This Population II star is the unconfirmed oldest star observed in the Universe. The oldest known star is SMSS J031300.36-670839.3 and it formed approximately 13.6 Bya. It is a star in the constellation Hydrus and is 6,000 light years from Earth.
Around 300 million years after the Big Bang (13.499 Bya) the first large-scale astronomical objects, such as protogalaxies and quasars are thought to have begun forming. During this time period Population III (Pop III) stars continued to burn and stellar nucleosynthesis operated. At first they were fusing hydrogen to produce more helium, then over time these Pop III stars were forced to fuse helium to produce carbon, oxygen, silicon and other heavy elements up to iron on the periodic table.
These new elements seeded into neighbouring gas clouds by the supernova of Pop III stars. This in turn led to the formation of more Population II stars (metal poor) and gas giants in the early universe through gravitational collapse.
It is also thought that Population II stars through their own nuclear fusion and supernovas created all the other elements in the periodic table, except the more unstable ones. An interesting characteristic of Population II (Pop II) stars is that despite their lower overall metallicity, they often have a higher ratio of alpha elements (O, Si, Ne, etc.) relative to Fe as compared to Population I stars.
Protogalaxy (Primeval Galaxy) is the cloud of gas, which forms into a galaxy. It is believed that the rate of star formation during this period of galactic evolution will determine whether a galaxy is a spiral or elliptical galaxy. A slower star formation tends to produce a spiral galaxy. The smaller clumps of gas in a Protogalaxy form into stars. Population II (Pop II) [and much later Population I (Pop I)] stars would have formed in the bulge of these galaxies.
380 million years after the Big Bang (13.419 Bya) the oldest known quasar (UDFj-39546284) formed in the constellation Fornax.
A Quasar (QSO or quasi-stellar object) is an extremely luminous active galactic nucleus (AGN). It has been theorized that most large galaxies contain a super massive central black hole with mass ranging from millions to billions of times the mass of our Sun.
In quasars and other types of AGN, the black hole is surrounded by a gaseous accretion disk. As gas falls toward the black hole, energy is released in the form of electromagnetic radiation, which can be observed across the electromagnetic spectrum. The power radiated by quasars is enormous. The most powerful quasars have luminosities thousands of times greater than a galaxy such as the Milky Way.
400 million years after the Big Bang (13.399 Bya) GN-z11, the oldest-known galaxy, formed. GN-z11 is a high-redshift (z = 11.09+0.08; −0.12) galaxy found in the constellation Ursa Major.
Roughly 400 million years after the Big Bang (13.399 Bya), the universe began to come out of its Dark Age due to the process of reionization slowing and the clearing of foggy hydrogen gas. The Population III stars generation had ended in supernovas as they had finished burning their hydrogen fuel. The Population II stars were now dominating the early universe. The early universe started to become transparent to ultraviolet light for the first time. Due to mass star formation the early universe starts to heat once again.
Around 400 to 700 million years after the Big Bang (13. 399 to 13.099 Bya) Galaxy Clusters and Superclusters started to emerge.
A Galaxy Cluster (cluster of galaxies) is a structure that consists of anywhere from hundreds to thousands of galaxies that are bound together by gravity with typical masses ranging from 1014–1015 solar masses.
A Supercluster is a large group of smaller galaxy clusters or galaxy groups. They are among the largest-known structures of the cosmos. The Milky Way is part of the Local Group galaxy group, which contains more than 54 galaxies, which in turn is part of the Laniakea Supercluster.
420 million years after the Big Bang (13.379 Bya) the quasar MACS0647-JD, (the furthest known quasar), formed. It is a formation of the MACS0647-JD high redshift (z = 10.7 – 11) galaxy in the constellation Camelopardalis.
599 million years after the Big Bang (13.2 Bya) EGSY8p7 a high redshift (z = 8.68) galaxy formed in the constellation Boötes.
About 630 million years after the Big Bang (13.169 Bya) the oldest ever observed gamma ray burst (GRB 090423) occurred.
669 million years after the Big Bang (13.13 Bya) EGS-zs8-1 a high-redshift (z = 7.7) Lyman-break galaxy formed in the northern constellation of Boötes.
699 million years after the Big Bang (13.1 Bya) z8_GND_5296 a dwarf high-redshift (z = 7.5078) Lyman-alpha galaxy formed in the constellation Ursa Major.
889 million years after the Big Bang (12.91 Bya) SXDF-NB1006-2 a redshift (z = 7.213) galaxy formed in the Cetus constellation.
899 million years after the Big Bang (12.9 Bya) GN-108036 a redshift (z = 7.2) galaxy formed in the constellation Ursa Major.
919 million years after the Big Bang (12.88 Bya) IOK-1 a redshift (z = 6.96) galaxy formed in the constellation Coma Berenices.
The early universe gradually transitioned into our known observable universe as seen today. The Dark Age came to an end at about 1 billion years after the Big Bang (12.799 Bya) as the Reionization era ended due to the early universe becoming ionized.
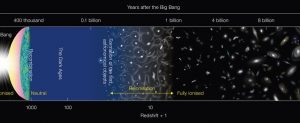
What Do You Think?
Was there a plan? Or was it a fluke?
The Dark Age lasted about 999,623 years, somewhere between 3.377 million years (13.795623 Bya) and 1 billion years (12.799 Bya) after the Big Bang. This was a miraculous period of darkness that laid the foundations for life!
The reionization was the silver lining of the dark gaseous clouds and the formation of stars and quasars provided light at the end of the dark tunnel of the early universe.
Our early universe has gone through its embryonic stage and is entering its fetus stage after struggling to survive. A wondrous development is soon to take place.